Last Updated: October 24, 2024
Introduction to the Metabolic Syndrome
Several years ago Dr. Gerald M. Reaven put forth the concept that the insulin resistance syndrome was the root cause of glucose intolerance, elevated LDL along with reduced HDL, and hypertension. This clinical concept has evolved into what is now referred to as the metabolic syndrome. The metabolic syndrome, MetS (also once referred to as Syndrome X), is a disorder that defines a combination of metabolic and cardiovascular risk determinants. These risk factors include insulin resistance, hyperinsulinemia, central adiposity (obesity associated with excess fat deposits around the waist), dyslipidemia, glucose intolerance, hypertension, pro-inflammatory status, and microalbuminemia.
The hallmark feature of MetS is indeed insulin resistance. Several other clinical abnormalities have recently been associated with MetS including non-alcoholic fatty liver disease (NAFLD), atherosclerosis, oxidative stress, and polycystic ovary syndrome (PCOS). It should be noted that NAFLD is now referred to as metabolic dysfunction-associated fatty liver disease (MAFLD).
Although obesity, ectopic fat accumulation, and an inflammatory status are central to the pathology of MetS, not all obese individuals develop MetS and not all individuals with MetS are obese. These observations indicate that MetS has a multifactorial etiology that involves a series of complex interactions between a particular individuals dietary habits, hormonal status, and genetic background. Increasing evidence indicates that the risk of MetS can be developmentally induced. Epidemiological studies in humans and animal models of MetS demonstrate an association between poor nutrition during fetal development and an increased risk of adult cardiovascular disease.
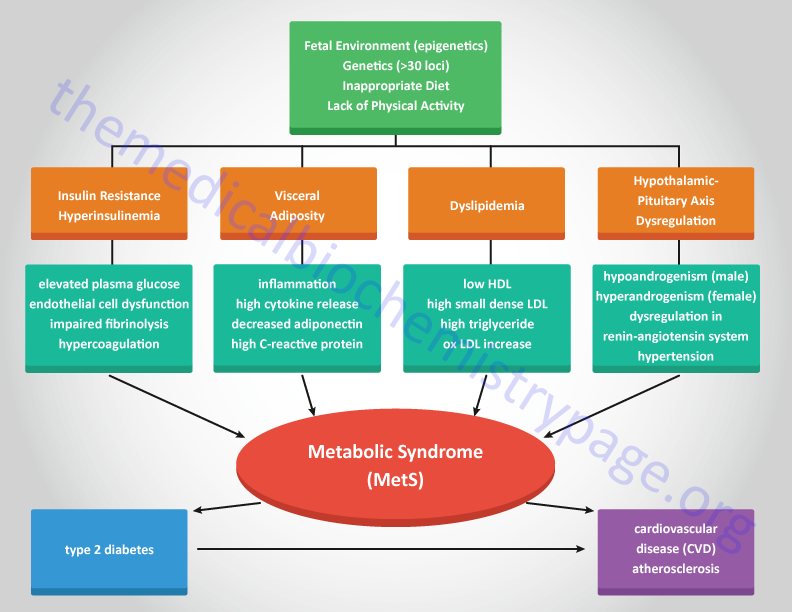
Given the complexities of the factors contributing to the metabolic syndrome numerous health groups in various countries have defined the disorder with slightly different criteria. Some health organizations believe that insulin resistance is the single most important predictor for future development of type 2 diabetes and cardiovascular disease that the disorder is defined as the insulin resistance syndrome. In addition, there are significant differences in ethnic predisposition to the effects of the dysfunctions of the metabolic syndrome that some criteria must be assessed with this in mind. Of particular note is the use of waist circumference as an indicator of obesity. The following Table lists the criteria set forth by the American Heart Association and the National Heart, Lung and Blood Institute as defining the metabolic syndrome.
Table of Criteria for Diagnosis of the Metabolic Syndrome
Defining Criteria | Parameters of Criteria |
elevated fasting blood glucose | ≥ 100 mg/dL (≥ 5.6mmol/L) |
elevated waist circumference | ≥ 102 cm (≥ 40 inches) in men ≥ 88cm (≥ 35 inches) in women |
elevated triglycerides | ≥ 150mg/dL (≥ 1.7mmol/L) |
reduced HDL cholesterol (HDLc) | < 40mg/dL (< 1.03mmol/L) in men < 50mg/dL (< 1.3mmol/L) in women |
elevated blood pressure | ≥ 130mm Hg systolic or ≥ 85mm Hg diastolic |
Genetic Factors in Metabolic Syndrome
Numerous epidemiological studies have implicated developmental origins in the progression to the metabolic syndrome. There appears to be a clear correlation between poor nutritional status of the mother during fetal development and the potential for increased risk for MetS to her child in later life. These correlations have also been observed in animal studies where prenatal diets in mothers impacts their offspring. Overfeeding of the mother during fetal development as well as excessive nutritional intake by the mother during the pre-weaning period of postnatal development results in increased obesity, adipocyte hypertrophy, reduced activity, insulin resistance, elevated blood pressure, endothelial cell dysfunction, and altered cardiovascular and renal function in offspring. When mothers are obese and fed high-fat diets during fetal development their adult offspring exhibit impaired glucose tolerance, hyperinsulinemia, dyslipidemia, hypertension, resistance to the anorexic actions of the leptin on the hypothalamus, and develop MAFLD. Additional evidence indicates that manipulation of fetal protein access leads to modified pancreatic islet cell expansion resulting in reduced pancreatic β-cell mass and persistent deficits in glucose homeostasis.
There is now a clear link between maternal dietary status and future development of MetS in adult offspring. Likewise, because the risks of metabolic syndrome in the general population, due to identified inherited polymorphisms is relatively small, the huge increase in the numbers of individuals with the disorder is most likely due to epigenetic changes that occur during early fetal development. The role of epigenetics in transgenerational disease manifestation has been outlined in several studies on the outcomes of children born to parents who experienced famine while pregnant. These children are far more likely to develop diabetes, obesity, and cardiovascular disease than children from parents of similar backgrounds who were not nutritionally deprived.
Of striking significance is that the second generation children (grandchildren of the starved mothers) were more likely to be born with low birth weight regardless of the nutritional status of their mothers. The potential for diet and nutrition to effect epigenetic changes in offspring on multiple generations has been conclusively demonstrated in animal models. In mice, the lack of adequate fetal nutrition results in a reduction in the methylation status of the promoter regions of several transcriptional regulators.
Hypomethylation of genes is most often associated with increased transcriptional activation. One transcriptional regulator of key metabolic significance whose promoter has been found to be hypomethylated in offspring of nutritionally deprived mothers is PPARα. PPARα is highly expressed in the liver, skeletal muscle, heart, and kidney. Its function in the liver is to induce hepatic peroxisomal fatty acid oxidation during periods of fasting. Expression of PPARα is also seen in macrophage foam cells and vascular endothelium. Its role in these cells is thought to be the activation of anti-inflammatory and anti-atherogenic effects. For a detailed discussion of the PPAR family of transcriptional regulators go to the Peroxisome Proliferator-Activated Receptors, PPARs page.
The recent use of genome-wide association studies (GWAS), in large groups of individuals, has allowed for the identification of genetic polymorphisms (single nucleotide polymorphisms, SNP) that are correlated increased risk for development of MetS. Several of these genes, as well as their normal functions, are described in the following Table.
Table of Genetic Polymorphisms Associated with Development of MetS
Gene Name | Gene Symbol | Comments |
anti-Müllerian hormone receptor type 2 | AMHR2 | receptor for anti-Müllerian hormone; upon ligand binding the receptor regulates folliculogenesis |
BAF chromatin remodeling complex subunit BCL7B | BCL7B | involved in cell cycle progression, maintenance of the nuclear structure, and stem cell differentiation; involved in the regulation of Wnt signaling |
centromere protein X | CENPX | involved in mitotic progression; DNA-binding component of the Fanconi anemia (FA) core complex that responds to DNA damage; critical in cellular responses to DNA cross-linking drugs |
fasciculation and elongation protein zeta 2 | FEZ2 | involved in axonal outgrowth and fasciculation (the process by which axons bundle together into nerve tracts) |
histocompatibility minor 13 | HM13 | required to generate lymphocyte cell surface epitopes derived from MHC class I signal peptides |
mediator complex subunit 23 | MED23 | subunit of the Mediator (MED) complex, specifically the tail module; MED complex plays a critical role as a coactivator for RNA polymerase II-mediated transcription of mRNAs |
carbohydrate response element-binding protein (ChREBP) | MLXIPL | ChREBP is also identified as MLX interacting protein like; is a basic helix-loop-helix (bHLH) leucine zipper (LZ) transcription factor of the Myc/Max/Mad superfamily; functions as a glucose responsive transcriptional regulator of genes involved in glycolysis, the pentose phosphate pathway, gluconeogenesis, and fatty acid synthesis |
myosin 1F | MYO1F | member of the myosin protein family of actin-based motor molecules with ATPase activity |
RNA binding motif protein 5 | RBM6 | RNA binding protein that promotes homologous recombination repair of double-strand breaks; modulates sensitivity to chemotherapeutic drugs |
RFT1 (Requiring Fifty-Three protein 1) homolog | RFT1 | functions as an intramembrane glycolipid transporter; catalyzes the translocation of the Man5GlcNAc2-PP-Dol intermediate from the cytoplasmic to the luminal side of the endoplasmic reticulum (ER) membrane in the pathway for the N-glycosylation of proteins; mutations in RFT1 gene are the cause of congenital disorder of glycosylation 1N (CDG1N) |
Sp1 (specificity protein 1) transcription factor | SP1 | involved in the regulation of the expression of genes required for the progression of the cell cycle and entry into S-phase |
Metabolic Disruptions in Metabolic Syndrome
Although MetS is not exclusively associated with type 2 diabetes and the associated insulin resistance, the increasing prevalence of obesity and associated development of type 2 diabetes places insulin resistance as a major contributor to the syndrome. As indicated above, the metabolic syndrome is defined as a clustering of atherosclerotic cardiovascular disease risk factors that include visceral adiposity (obesity), insulin resistance, low levels of HDLs, and a systemic proinflammatory state. There are key components to the metabolic syndrome which include, in addition to insulin resistance (the hallmark feature of the syndrome), hypertension, dyslipidemia, chronic inflammation, impaired fibrinolysis, procoagulant state, and central obesity.
The role of adipose tissue (fat) stems from the fact that the organ is active at secretion of cytokines, termed adipocytokines. These include tumor necrosis factor-α (TNFα), interleukin-6 (IL-6), leptin, adiponectin, and resistin. Leptin has received particular attention of late due to its role in obesity in addition to the fact that recent data indicates that plasma leptin levels are found to be predictive of the potential for cardiovascular pathology.
Many clinicians and researchers believe that insulin resistance underlies the cardiovascular pathologies of the metabolic syndrome. One primary reason for this is the role of insulin in fat homeostasis. As indicated above, the major role of insulin is to induce the storage of fuel. This can be as fat (triglycerides, TG) in adipose tissue or as carbohydrate in the form of glycogen in liver and skeletal muscle. The effect of insulin resistance at the level of fat homeostasis is an increase in circulating TG, referred to as dyslipidemia. Due to insulin resistance there is an increase in the delivery of peripheral fatty acids to the liver which in turn drives hepatic TG synthesis. These TG are then packaged into lipoprotein particles termed VLDL (very low density lipoproteins) which are returned to the circulation.
An additional role of insulin resistance in the overall cardiac pathology associated with the metabolic syndrome relates to the normal role of insulin in platelet function. In platelets, insulin action leads to an increase in endothelial nitric oxide synthase (eNOS) activity that is due to its phosphorylation by AMPK. Activation of NO production in platelets leads to a decrease in thrombin-induced aggregation, thereby, limiting the pro-coagulant effects of platelet activation. This response of platelets to insulin function clearly indicates why disruption in insulin action is a major contributing factor in the development of the metabolic syndrome.
Taken together, the insulin resistance and its associated negative effects on metabolism, the increased levels of circulating TG, the reduced levels of HDL and hypertension, all contribute to the progression of atherosclerosis. With associated coagulation and fibrinolysis pathologies, the cardiovascular events of the metabolic syndrome can be devastating. Since many of these pathologies can be reversed with proper diet and exercise, it is in a persons’ best interest to take responsibility for the role their life style choices play in the development of the metabolic syndrome.
Obesity and the Metabolic Syndrome
Although, as indicated above, there are many examples of individuals who are obese but do not manifest MetS there is still a very strong correlation between obesity and increased risk for development of MetS. In addition, the incidence of MetS increases with the severity of obesity. It has been observed that over 50% of obese adolescents go on to develop MetS. Also, given the high correlation between MetS and insulin resistance it is not at all surprising that there is a strong link between obesity and MetS. Insulin resistance (identified as type 2 diabetes) is 5 to 6 times more common in individuals with a body mass index (BMI) >30kg/m2 than in individuals of normal weight.
Although the precise mechanism(s) by which obesity leads to the development of MetS is not fully understood, there are many overlapping pathways of metabolism that are disrupted in obesity that can be identified as important in the progression to MetS. Not the least of which is the role that obesity, in particular visceral obesity, plays in the progression to insulin resistance. Recall that as mentioned above, insulin resistance is the hallmark manifestation of MetS.