Last Updated: October 11, 2024
Introduction to the Eicosanoids
The principal eicosanoids (from Ancient Greek eíkosi for 20) of biological significance to humans are a group of bioactive lipid molecules derived from the polyunsaturated fatty acid (PUFA), arachidonic acid. Arachidonic acid is a 20 carbon fatty acid containing four sites of unsaturation (20:4). These eicosanoids primarily consist of the prostaglandins (PG), thromboxanes (TX), leukotrienes (LT), and lipoxins (LX). The PG and TX are collectively identified as prostanoids.
Prostaglandins were originally shown to be synthesized in the prostate gland, thromboxanes from platelets (thrombocytes), and leukotrienes from leukocytes, hence the derivation of their names.
The prostaglandins, thromboxanes, leukotrienes, and lipoxins belong to a large family of bioactive lipids referred to as the oxylipins. Oxylipins are derived from omega-6 and omega-3 PUFA including the essential fatty acids. The various oxylipins are synthesized via the actions of cyclooxygenases, lipoxygenases, and cytochrome P450 (CYP) enzymes.
The lipoxins are inflammation resolving eicosanoids synthesized through lipoxygenase interactions (hence the derivation of the name). Lipoxins are potent inflammation modulating eicosanoid derivatives and their synthesis can be increased in response to ingestion of aspirin (see the Bioactive Lipid Mediators of Inflammation page for more details on lipoxin functions). Additional inflammation modulating lipid compounds, whose synthesis can also be triggered by aspirin, are the resolvins (Rv) and the protectins (PD) and these are also discussed in the Bioactive Lipid Mediators of Inflammation page.
In addition to the four classes of oxylipins, that are derived from arachidonic acid, there are the resolvins, protectins (also called neuroprotectins in the brain), maresins, mono- and dihydroxy fatty acids, epoxy fatty acids, eoxins, and hepoxilins.
Additional important arachidonic acid-derived bioactive lipids include adrenic acid and the omega-6 form of docosapentaenoic acid (DPAn-6). Adrenic acid is all-cis-7,10,13,16-docosatetraenoic acid (DTA). DPAn-6 is all-cis-4,7,10,13,16-docosapentaenoic acid (trivial name: osbond acid).
Eicosanoid Nomenclature
The nomenclature of the eicosanoids includes a subscript number which refers to the number of carbon-carbon double bonds that exist in the molecule. The majority of the biologically active prostaglandins and thromboxanes are referred to as series 2 molecules due to the presence of two carbon-carbon double bonds. The predominant leukotrienes are series 4 molecules due to the presence of four carbon-carbon double bonds. There are, however, important series 1 prostaglandins and thromboxanes as described below.
Confusion can result in the classification of eicosanoids due to the use of the term “group” to define the origin of the various eicosanoids.
Group 1 eicosanoids are all derived from dihomo-γ-linolenic acid (DGLA; a 20:3 omega-6 PUFA) which is an intermediate in the synthesis of arachidonic acid from the essential fatty acid, linoleic acid, as outlined in the Figure below. DGLA is a substrate for COX-1 and two lipoxygenases, 12-LOX and 15-LOX. DGLA serves as the substrate for the synthesis of PGD1, PGE1, PGF1α, and TXA1 as well as the platelet-derived 12(S)-hydroperoxy-8,10,14-eicosatrienoic acid [12(S)-HpETrE].
Group 2 eicosanoids are all derived from arachidonic acid (a 20:4 omega-6 PUFA). Arachidonic acid is the substrate for the synthesis of a wide array of bioactive lipids as described in more detail in the sections below. The major pro-inflammatory and/or pro-thrombotic eicosanoids derived from arachidonic acid include PGE2, TXA2, and LTB4.
Group 3 eicosanoids are all derived from eicosapentaenoic acid (EPA; a 20:5 omega-3 PUFA) which itself is synthesized from the essential fatty acid, α-linolenic (ALA). PGD3 and PGI3 decrease platelet aggregation and they counter the effects of PGD2.
The letters (A through J) that define the various prostaglandins, such as PGE2, describe the placement and stereochemistry of the different functional groups that are attached to the ring. Thromboxanes are lettered A or B according to the type of ring structure that is in the molecule. The leukotrienes are labeled A if they are 5(6) epoxides and B if they are dihydroxy compounds. The C, D, or E nomenclature for the leukotrienes refers to them being peptidolipids (amino acid modified; also referred to as peptidoleukotrienes).
Representative Clinically Relevant Eicosanoids
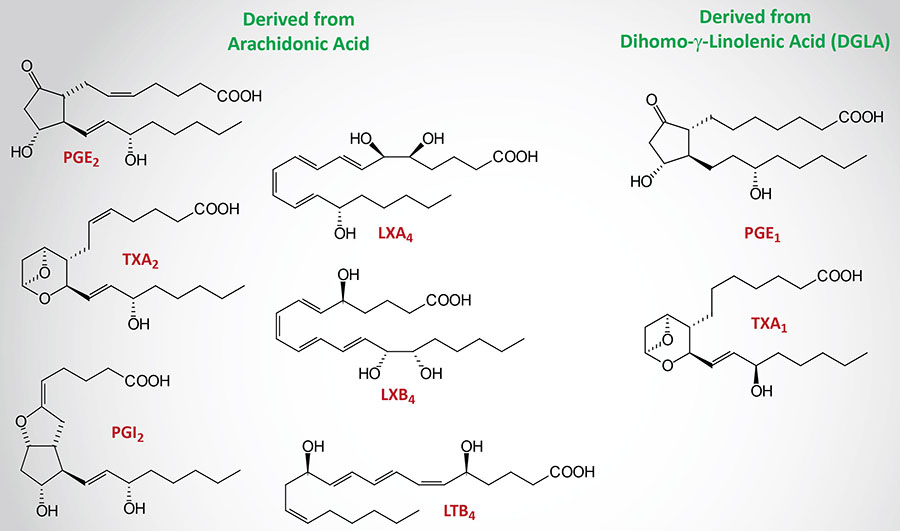
The eicosanoids produce a wide range of biological effects on inflammatory responses (predominantly those of the joints, skin and eyes), on the intensity and duration of pain and fever, and on reproductive function (including the induction of labor). They also play important roles in inhibiting gastric acid secretion, regulating blood pressure through vasodilation or constriction, and inhibiting or activating platelet aggregation and thrombosis.
As indicated, the principal eicosanoids of biological significance to humans are derived from arachidonic acid. Additional biologically significant eicosanoids are derived from dihomo-gamma(γ)-linolenic acid (DGLA) which is produced in the reaction pathway leading to arachidonic acid from the essential fatty acid, linoleic acid (see Figure below). Other important eicosanoids are derived from the omega-3 fatty acids, eicosapentaenoic acid (EPA) and docosahexaenoic acid (DHA), which are derived from the essential fatty acid, alpha(α)-linolenic acid (ALA), or obtained in the diet.
The major source of arachidonic acid is through its release from membrane phospholipids. Within cell membranes, arachidonic acid resides predominantly at the C–2 position of phospholipids (primarily phosphatidylinositol-4,5-bisphosphate, PIP2) and is released from there upon the activation of enzymes of the phospholipase A2 (PLA2) family. Go to the Signal Transduction: Phospholipids page for details on the various PLA2 enzymes.
Synthesis of Arachidonic Acid
The immediate dietary precursor of arachidonic acid is the 18-carbon essential fatty acid, linoleic acid. Linoleic acid is converted to arachidonic acid through the three reaction steps outlined in the Figure below. The activity of the Δ6-desaturase (D6D; encoded by the FADS2 gene) is slow and can be further compromised due to nutritional deficiencies as well as during inflammatory conditions. Therefore, maximal capacity for synthesis of arachidonic acid occurs with ingested gamma(γ)-linolenic acid (GLA), the product of the Δ6-desaturase reaction.
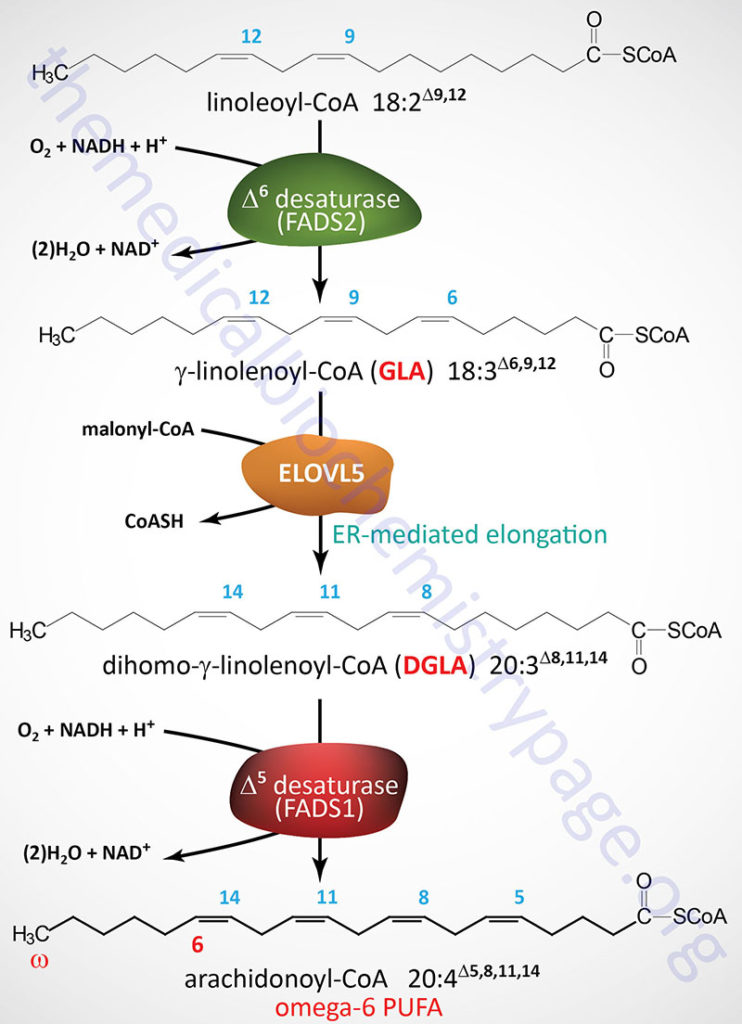
The D6D enzyme is officially called fatty acid desaturase 2 which is encoded by the FADS2 gene. The FADS2 gene is located on chromosome 11q12.2 and is composed of 13 exons that generate three alternatively spliced mRNAs each of which encode distinct protein isoforms.
GLA is converted to dihomo-γ-linolenic acid (DGLA) via the microsomal (ER) fatty acid elongation pathway. The enzyme involved in this elongation step is encoded by the ELOVL5 (elongation of very long-chain fatty acids protein 5) gene. The ELOVL5 gene is located on chromosome 6p12.1 and is composed of 10 exons that generate five alternatively spliced mRNAs that collectively encode four distinct protein isoforms.
DGLA is subsequently further unsaturated to arachidonic acid by the enzyme Δ5-desaturase (D5D). The D5D enzyme is officially called fatty acid desaturase 1 which is encoded by the FADS1 gene. The FADS1 gene is located very near to the FADS2 gene on chromosome 11q12.2 and is composed of 13 exons that encode a protein of 501 amino acids.
Like the Δ6-desaturase, the activity of the Δ5-desaturase is limiting in arachidonic acid synthesis and its activity is also influenced by diet and environmental factors. The activity of the D5D and D6D enzymes is also compromised by the consumption of pro-inflammatory omega-6 fatty acids, such as the essential fatty acid linoleic acid and arachidonic acid, as is typical in the Western-style diet. Consumption of alcohol has also been shown to reduce the level of D6D activity. In addition, hyperglycemia and hypercholesterolemia are both known to interfere with the activity of D5D and D6D.
Due to the limited activity of the Δ5-desaturase most of the DGLA formed from GLA is inserted into membrane phospholipids at the same C-2 position as for arachidonic acid. As discussed in detail below, DGLA serves as a precursor for the series-1 eicosanoids which exert opposing biological effects to the series-2 eicosanoids derived from arachidonic acid.
The major dietary sources of GLA are borage oil, evening primrose seed oil, hemp seed oil, and black currant seed oil. Diets containing sources of GLA have been shown have distinct cardiovascular benefit similar to diets rich in omega-3 polyunsaturated fatty acids (PUFA) such as is found in cold water fishes (e.g. salmon, mackerel, and sardines), Krill oils, algae, and seaweed. Outside the context of fortified foods, algae are the best natural sources of EPA and DHA for vegetarians and vegans.
Arachidonic Acid-Derived Bioactive Lipids
Through the actions of various cyclooxygenases, lipoxygenases, and CYP enzymes, arachidonic acid is converted to a vast array of bioactive lipids that are collectively identified as oxylipins. As indicated in the introduction, these arachidonic acid-derived bioactive lipids include the prostaglandins, thromboxanes, leukotrienes, and lipoxins.
Additional bioactive arachidonic acid-derived oxylipins are the epoxides, the ω-hydroxylated hydroxyeicosatetraenoic acids (HETE), the hepoxilins, and the lipoxygenase-derived hydroxyheptadecatrienoic acids (HpETE) and HETE.
The lipoxygenase pathway for arachidonic acid-derived 15-HpETE are precursors for the synthesis of the lipoxins, whereas the aspirin-modified COX-2 pathway results in the synthesis of the epi-lipoxins.
Eicosanoid Synthesis
All mammalian cells except erythrocytes synthesize eicosanoids. These molecules are extremely potent, able to cause profound physiological effects at very dilute concentrations. All eicosanoids function locally at the site of synthesis, through receptor-mediated G-protein linked signaling pathways.
Two main pathways are involved in the biosynthesis of eicosanoids, the cyclic and the linear pathways. The prostaglandins and thromboxanes are synthesized by the cyclic pathway, the leukotrienes and lipoxins are synthesized by the linear pathway.
Prostaglandin and Thromboxane Synthesis
The cyclic pathway of eicosanoid synthesis is initiated through the action of prostaglandin G/H synthase, PTGS (also called prostaglandin-endoperoxide synthetase). This enzyme possesses two activities, cyclooxygenase (COX) and peroxidase. There are two forms of the PTGS activity in humans most commonly identified as COX-1 (PTGS-1) and COX-2 (PTGS-2). Both COX-1 and COX-2 catalyze the two-step conversion of arachidonic acid to PGG2 and then to PGH2.
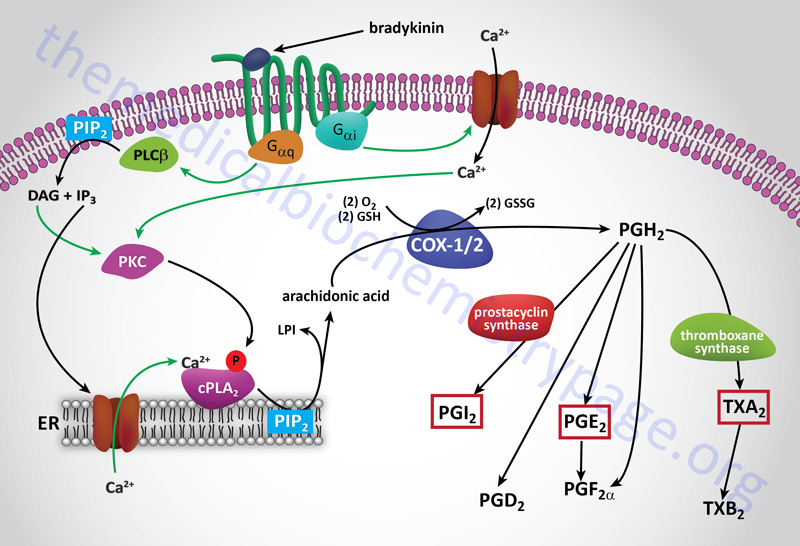
COX and the Synthesis of PGH2
Arachidonic acid is converted to PGH2 in a two-step reaction pathway involving the actions of the bi-functional enzymes COX-1 (PTGS-1) and COX-2 (PTGS-2). These enzymes are also called prostaglandin G/H synthase (PTGS) or prostaglandin endoperoxide synthetase. Arachidonic acid is first converted to PGG2 and then to PGH2.
COX-1 (PTGS-1) is expressed constitutively in gastric mucosa, kidney, platelets, and vascular endothelial cells. The primary eicosanoids generated via the COX-1 pathway are PGD2 and TXA2. The COX-1 derived eicosanoids modify vascular tone, increase platelet aggregation, and are involved in immune surveillance.
COX-2 (PTGS-2) is inducible and is expressed in macrophages and monocytes in response to inflammation and is also highly expressed in the brain and the kidneys. The primary triggers for COX-2 induction in monocytes and macrophages are platelet-activating factor, PAF and interleukin-1, IL-1.
The primary eicosanoids generated via the COX-2 pathway are PGE2, prostacyclin (PGI2), PGD2, and PGF2α. The eicosanoids of the COX-2 pathway are involved in immune responses, regulation of vessel tone, nociception (sensation of pain), formation of blood clots, and female fertility.
COX-1 is encoded by the PTGS1 gene. The PTGS1 gene is located on chromosome 9q33.2 and is composed of 14 exons that generates seven alternatively spliced mRNAs that collectively encode six protein isoforms. One of the alternatively spliced PTGS1 derived mRNAs encodes a cyclooxygenase enzyme identified as COX-3. The expression of COX-3 is reportedly higher in the small vessels of the brain and heart.
COX-2 is encoded by the PTGS2 gene. The PTGS2 gene is located on chromosome 1q31.1 and is composed of 10 exons that encode a precursor protein of 604 amino acids.
Synthesis of Prostacyclin: PGI2
Prostacyclin (PGI2) is synthesized from PGH2 via the action of prostacyclin synthase (encoded by the PTGIS gene). Prostaglandin synthase is a member of the large family of cytochrome P450 (CYP) enzymes and as such is also identified as CYP8A1.
The PTGIS gene is located on chromosome 20q13.13 and is composed of 11 exons that encode a 500 amino acid precursor protein.
Synthesis of PGE2
PGE2 is synthesized from PGH2 via the action of one of several PGE synthases, encoded by the PTGES, PTGES2, and PTGES3 genes. The PGE synthase (encoded by the PTGES gene) appears to be the key enzyme in overall PGE2 synthesis.
The PTGES gene is located on chromosome 9q34.11 and is composed of 3 exons that encode a 152 amino acid protein. The PTGES encoded enzyme is also identified as mPGES-1 for membrane-associated PGE synthase 1.
The PTGES2 encoded enzyme is also identified as mPGES-2 for membrane-associated PGE synthase 1.
The PTGES3 encoded enzyme is also identified as cPGES for cytosolic PGE synthase.
Synthesis of TXA2
The principal thromboxane (TXA2) is derived from PGH2 via the action of thromboxane A synthase 1 encoded by the TBXAS1 gene. Thromboxane A synthase 1 is a member of the large family of cytochrome P450 (CYP) enzymes and as such is also identified as CYP5A1.
The TBXAS1 gene is located on chromosome 7q34 and is composed of 20 exons that generate eight alternatively spliced mRNAs that collectively encode seven different protein isoforms. The formation of TXB2 results from degradation of TXA2 and is known to play a role in the hepatotoxicity of acetaminophen.
Synthesis of PGD2
Two forms of PGD2 synthase have been identified that convert PGH2 to PGD2. One is encoded by the HPGDS (hematopoietic prostaglandin D synthase) gene and the other is encoded by the PTGDS (prostaglandin D2 synthase) gene. The enzyme encoded by the HPGDS gene is a member of the large family of cytosolic glutathione S-transferase enzymes.
The HPGDS gene is located on chromosome 4q22.3 and is composed of 6 exons that a 199 amino acid protein. The HPGDS encoded enzyme is also referred to as hematopoietic prostaglandin D synthase, H-PGDS. The HPGDS encode enzyme is a member of the cytosolic glutathione-S-transferase (GST) family of enzymes and in this family is identified as sigma GST.
The PTGDS gene is located on chromosome 9q34.3 and is composed of 7 exons that encode a 190 amino acid precursor protein. The PTGDS encoded enzyme is also referred to as lipocalin-type prostaglandin D synthase, L-PGDS.
Synthesis of PGD2 from PGH2 can also be catalyzed by an enzyme of the aldo-keto reductase 1 family, specifically the enzyme encoded by the AKR1B1 (aldo-keto reductase family 1 member B) gene.
Synthesis of PGF2α
PGF2α possesses two hydroxyl groups, one on the 9-position and one on the 11-position, which allows for four stereoisomeric forms of this prostaglandin. These stereoisomers include 9α-,11α-PGF2 (PGF2α), 9α-,11β-PGF2 (11-epi PGF2α), 9β-,11α-PGF2 (9-epi PGF2α), and 9β-,11β-PGF2 (9-,11-epi PGF2α). Of these four stereoisomers, two are synthesized in vivo in human cells, PGF2α, and 9α-,11β-PGF2.
The synthesis of PGF2α from PGH2 is catalyzed by the enzyme aldo-keto reductase family 1 member C3 which is encoded by the AKR1C3 gene. The AKR1C3 encoded enzyme is also known as prostaglandin F synthase.
The AKR1C3 encoded enzyme can also convert PGD2 to 9α-,11β-PGF2.
The AKR1C3 gene is located on chromosome 10p15.1 and is composed of 10 exons that generate three alternatively spliced mRNAs, each of which encode a distinct protein isoform.
PGE2 can also serve as a substrate for the synthesis of PGF2α. This reaction is catalyzed by the enzyme identified as prostaglandin 9-ketoreductase (PG-9-KR) which is encoded by the CBR1 (carbonyl reductase 1) gene.
Leukotriene Synthesis
The linear pathway of leukotriene synthesis is initiated through the action of members of the arachidonate lipoxygenase (LOX) family of enzymes. Humans express six genes encoding LOX enzymes identified as ALOXE3, ALOX5, ALOX12, ALOX12B, ALOX15, and ALOX15B.
The leukotrienes are synthesized by several different cell types including white blood cells (leukocytes, hence the derivation of the name of the compounds), mast cells, lung, spleen, brain, and heart.
ALOXE3 encodes arachidonate lipoxygenase 3 which is commonly designated E-LOX.
ALOX5 encodes arachidonate 5-lipoxygenase which is commonly designated 5-LOX. The ALOX5 gene is located on chromosome 10q11.21 and is composed of 14 exons that generate five alternatively spliced mRNAs each of which encode a distinct protein isoform.
ALOX12 encodes arachidonate 12-lipoxygenase, 12S type which is commonly designated 12-LOX. The ALOX12 gene is located on chromosome 17p13.1 and is composed of 14 exons that encode a protein of 663 amino acids.
ALOX12B encodes arachidonate 12-lipoxygenase, 12R type which is commonly designated 12R-LOX.
ALOX15 encodes arachidonate 15-lipoxygenase which is commonly designated 15-LOX. The ALOX15 gene is located on chromosome 17p13.2 and is composed of 14 exons that encode a protein of 662 amino acids.
ALOX15B encodes arachidonate 15-lipoxygenase, type B which is commonly designated 15-LOX-2.
The lipoxygenases of leukotriene and lipoxin synthesis are 5-LOX, 12-LOX, and 15-LOX.
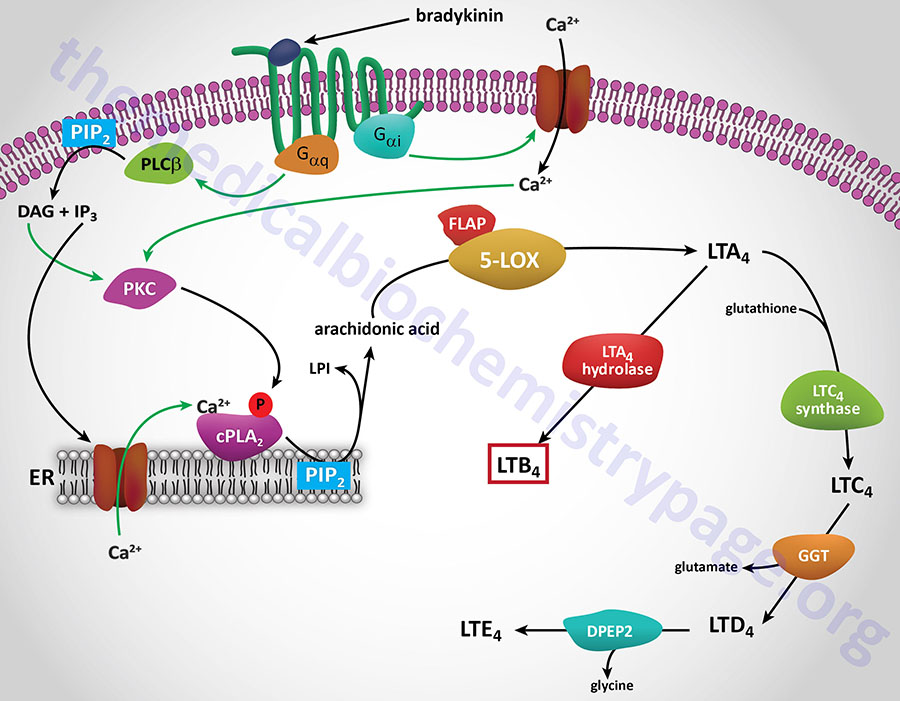
The ALOX5 encoded enzyme, 5-LOX, along with 5-LOX activating protein (FLAP), catalyzes the conversion of arachidonic acid to 5(S)-hydroperoxyeicosatetraenoic acid [5(S)-HpETE]. Without the aid of FLAP, 5-LOX converts 5(S)-HpETE to leukotriene A4 (LTA4). 5(S)-HpETE can spontaneously, or through the actions of a peroxidase, reduce to 5(S)-hydroxyeicosatetraenoic acid [5(S)-HETE].
LTA4 is then converted LTB4 and the cysteinyl leukotrienes as shown in the Figure above. LTA4 also serves as the precursor for the synthesis of the lipoxins, LXA4 and LXB4 as diagrammed in the Figure below as well as in the Bioactive Lipid Mediators of Inflammation page.
The synthesis of LTB4 from LTA4 involves the the enzyme identified as LTA4 hydrolase, encoded by the LTA4H gene. The LTA4H encoded enzyme is bifunctional possessing both hydrolase and aminopeptidase activities.
The conversion of LTA4 to LTC4, the precursor for the cysteinyl leukotrienes (peptidoleukotrienes), involves the action of an enzyme of the glutathione-S-transferase family commonly identified as leukotriene C4 synthase encoded by the LTC4S gene. LTC4 is then converted to LTD4 by an enzyme that is a member of the gamma-glutamyltransferase (GGT) family. LTD4 is converted to LTE4 by the enzyme dipeptidase 2 which is encoded by the DPEP2 gene.
The ALOX12 encoded enzyme, 12-LOX, converts arachidonic acid to 12(S)-hydroperoxyeicosatetraenoic acid [12(S)-HpETE] which then spontaneously reduces to 12(S)-HETE.
The ALOX15 encoded enzyme,15-LOX, converts arachidonic acid to 15(S)-hydroperoxyeicosatetraenoic acid [15(S)-HpETE]. 15(S)-HpETE is then converted to the lipoxins via the actions of 5-LOX.
Lipoxin Synthesis
The lipoxins are synthesized through the concerted actions of 15-LOX (acting on arachidonic acid in epithelial cells, such as in the airway) followed by 5-LOX in leukocytes or through the actions of 5-LOX in leukocytes followed by 12-LOX action in platelets. Details of the functions of the lipoxins can be found in the Bioactive Lipid Mediators of Inflammation page.
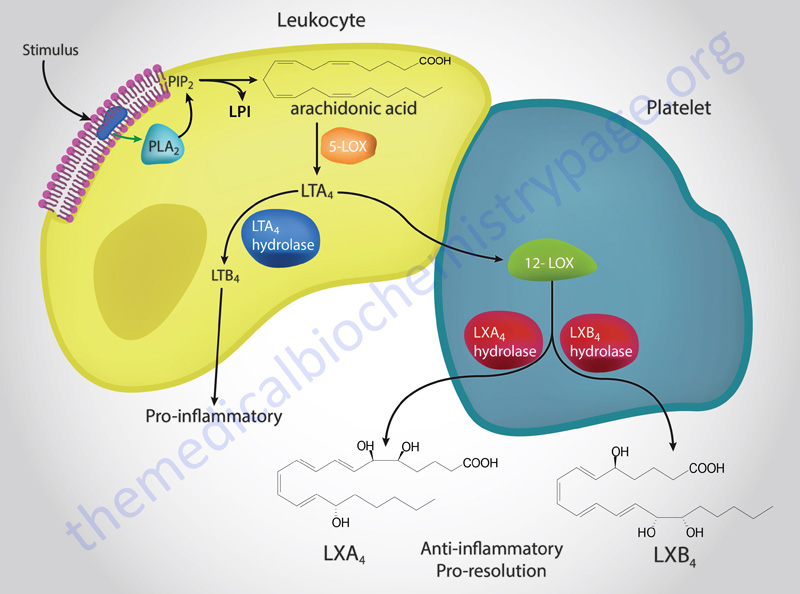
Eicosanoid Receptors
Each of the eicosanoids functions via interactions with cell-surface receptors that are members of the G-protein coupled receptor (GPCR) family. There are at least 13 characterized eicosanoid receptors that bind prostaglandins, thromboxanes, and leukotrienes. Receptors that bind the prostaglandin D family of lipids are called the DP receptors, those that bind E family prostaglandins are called the EP receptors, those that bind F family prostaglandins are called the FP receptors, those that bind prostacyclin (PGI2) are called the IP receptors, and those that bind the thromboxanes are called the TP receptors.
Prostaglandin D Family Receptors
Two members of the DP receptor family have been described, DP1 (DP1) and DP2 (DP2). The DP1 receptor is encoded by the PTGDR gene located on chromosome 14q22.1 and is composed of 4 exons that generate two alternatively spliced mRNAs. The DP1 receptor is coupled to the activation of a Gs-type G-protein.
The DP2 receptor is encoded by the PTGDR2 gene located on chromosome 11q12.2 and is composed of 2 exons the encode a protein of 395 amino acids. An earlier designation for the PTGDR2 gene was GPR44 as it was originally characterized as an orphan GPCR. Although the DP2 receptor does indeed bind PGD2 as a natural ligand, it is not related to the functional family (class A: rhodopsin) of the other prostanoid receptors but is a member of the chemoattractant (class B) receptor family. The DP2 receptor is coupled to the activation of a Gi-type G-protein.
Prostaglandin E Family Receptors
There are four members of the EP receptor family, EP1 (EP1), EP2 (EP2), EP3 (EP3), and EP4 (EP4).
The EP1 receptor is encoded by the PTGER1 gene located on chromosome 19p13.12 and is composed of 3 exons that encode a protein of 402 amino acids. The EP1 receptor is coupled to the activation of a Gq-type G-protein.
The EP2 receptor is encoded by the PTGER2 gene located on chromosome 14q22.1 and is composed of 2 exons that encode a protein of 358 amino acids. The EP2 receptor is coupled to the activation of a Gs-type G-protein.
The EP3 receptor is encoded by the PTGER3 gene located on chromosome 1p31.2 and is composed of 11 exons that generate at least 8 alternatively spliced mRNAs. The EP3 receptor has been shown to activate both Gi– and G12-type G-proteins.
The EP4 receptor is encoded by the PTGER4 gene located on chromosome 5p13.1 and is composed of 7 exons that encode a protein of 488 amino acids. The EP4 receptor is coupled to the activation of a Gs-type G-protein.
Thromboxane Receptor
The receptor for the thromboxanes is called the TP receptor. The TP receptor binds thromboxane A2 (TXA2) and it is encoded by the TBXA2R gene which is located on chromosome 19p13.3 and is composed of 5 exons that generate two alternatively spliced mRNAs, each of which encode distinct proteins, one of 343 amino acids (the α isoform) and one of 407 amino acids (the β isoform). The TP receptor is coupled to the activation of a Gq-type G-protein.
Prostacyclin Receptor
The receptor for prostacyclin (PGI2) is called the IP receptor. The IP receptor is encoded by the PTGIR gene which is located on chromosome 19q13.32 and is composed of 6 exons that encode a protein of 386 amino acids. The IP receptor couples to the activation of a Gs-type G-protein.
Prostaglandin F Receptor
The PGF2α receptor is identified as the FP receptor. The FP receptor is encoded by the PTGFR gene which is located on chromosome 1p31.1 and is composed of 7 exons that encode two alternatively spliced mRNAs both of which encode distinct protein isoforms. The FP receptor is coupled to the activation of a Gq-type G-protein.
Leukotriene Family Receptors
There are at least six receptor proteins that belong to the leukotriene receptor family. Two receptors have been characterized that bind LTB4 called BLTR (commonly identified as BLT1) and BLTR2 (commonly identified as BLT2). There are two receptors that bind the peptidoleukotrienes (cysteinyl leukotrienes) called CysLT1 and CysLT2. Another family member is the formyl peptide receptor 2 (originally identified as FPRL1. Another family member oxoeicosanoid receptor 1 which was originally identified as GPR170
The BLT1 receptor is encoded by the LTB4R gene. The LTB4R gene is located on chromosome 14q12 and is composed of 3 exons that generate two alternatively spliced mRNAs, both of which encode the same 352 amino acid protein. BLTR is coupled to a Gi-type G-protein. In addition to LTB4 the BLT1 receptor binds 20-hydroxy-LTB4 and 12(R)-hydroperoxyeicosapentaenoic acid [12(R)-HETE].
The BLT2 receptor is encoded by the LTB4R2 gene. The LTB4R2 gene is located on chromosome 14q12 and is composed of 2 exons generate two alternatively spliced mRNAs, both of which encode the same 358 amino acid protein. BLTR2 is coupled to both Gq– and Gi-type G-proteins. In addition to LTB4 the BLT2 receptor binds 12(S)-hydroperoxyeicosapentaenoic acid [12(S)-HETE].
The CysLT1 receptor is encoded by the CYSLTR1 gene. The CYSLTR1 gene is located on the X chromosome (Xq21.1) and is composed of 5 exons that generate four variant mRNAs, each of which encode the same 337 amino acid protein. CysLT1 is coupled to a Gi-type G-protein.
The CysLT2 receptor is encoded by the CYSLTR2 gene. The CYSLTR2 gene is located on chromosome 13q14.2 and is composed of 6 exons that generate seven alternatively spliced mRNAs, all of which encode the same 346 amino acids protein. CysLT2 is coupled to a Gq-type G-protein.
The formyl peptide receptor 2 is encoded by the FPR2 gene. The FPR2 gene is located on chromosome 19q13.41 and is composed of 3 exons that generate two alternatively spliced mRNAs encoding the same 351 amino acid protein. FPR2 is coupled to the activation of a Gi-type G-protein.
The oxoeicosanoid receptor 1 is encoded by the OXER1 gene. The OXER1 gene is an intronless gene located on chromosome 2p21 and encodes a 384 amino acid protein. Oxoeicosanoid receptor 1 is coupled to a Gi-type G-protein.
Eicosanoids and Inflammatory Responses
As indicated in the Table below, the major actions of the series-2 prostaglandins and thromboxanes (predominantly PGE2 and TXA2) are pro-inflammatory as are the series-4 leukotrienes (predominantly LTB4). Thus, it makes sense that drugs that reduce the production of these compounds would be beneficial at reducing inflammation and the associated vascular pathologies. A widely used class of drugs, the non-steroidal anti-inflammatory drugs (NSAIDs) such as ibuprofen, indomethacin, naproxen, and phenylbutazone all exert their effects on the cyclooxygenase activity, inhibiting both COX-1 (COX1) and COX-2 (COX2). Aspirin is unique among the class of NSAIDs in that its actions as an analgesic (relief from pain) and as an anti-inflammatory, as well as a heart protective drug, are not solely due to its ability to inhibit COX activity (go to the Bioactive Lipid Mediators of Inflammation page for details).
Research over the past 20-25 years has demonstrated the physiological benefits (i.e. anti-inflammatory; pro-resolution) of alternative pathways of polyunsaturated fatty acid metabolism. Much of this topic is covered in the Bioactive Lipid Mediators of Inflammation page. As described above for the synthesis of arachidonic acid, much of the DGLA derived from ingested linoleic acid or GLA is diverted into membrane phospholipids due to the inefficiency of the Δ5-desaturase catalyzing the conversion of DGLA to arachidonic acid. Incorporation of DGLA into membrane phospholipids competes with the incorporation of arachidonic acid so that diets enriched in GLA result in an alteration in the ratio of membrane arachidonic acid to DGLA. Release of membrane DGLA occurs through the action of PLA2 just as for release of arachidonic acid. Once DGLA is released it will compete with arachidonic acid for COX and LOX.
The products of COX action on DGLA are series-1 prostaglandins (PGE1) and thromboxanes (TXA1). These eicosanoids are structurally similar to the series-2 eicosanoids except, of course, they have a single double bond. Although structurally similar, the series-1 eicosanoids have distinctly different biological actions. PGE1 and TXA1 are anti-inflammatory, they induce vasodilation, and they inhibit platelet aggregation. When DGLA is a substrate for 15-LOX the product is 15-hydroxyeicosatrienoic acid (15-HETrE). 15-HETrE is a potent inhibitor of 5-LOX which is the enzyme responsible for the conversion of arachidonic acid to LTB4. LTB4 is a potent inflammatory molecule through its action on neutrophils, thus, DGLA serves to inhibit inflammation via the linear eicosanoid pathway as well.
Due to the vasodilating action of PGE1 it is used pharmaceutically as alprostadil for the treatment of erectile dysfunction (ED). The ED applications of PGE1 are sold as MUSE® and Caverject®. MUSE is a urethral suppository and Caverject is an injectable version. Alprostadil is also used clinically to treat newborn infants with ductal-dependent congenital heart disease. The administration of alprostadil in these infants maintains a patent ductus arteriosus until surgery can carried out to correct the underlying heart defect. Ductus arteriosus is a normal structure of the fetal heart that allows blood to bypass circulation to the lungs since the fetus does not use his/her lungs in utero. The ductus arteriosus shunts blood flow from the left pulmonary artery to the aorta. Shortly after birth the ductus closes due to the high levels of oxygen the newborn is exposed to at birth. However, in newborns with certain congenital heart defects, maintaining a patent ductus arteriosus is clinically significant.
Because inhibition of COX-1 activity in the gut is associated with NSAID-induced ulcerations, pharmaceutical companies have developed drugs targeted exclusively against the inducible COX-2 activity [e.g. Celebrex® (celecoxib), Prexige® (lumiracoxib) and the recently removed Vioxx® (rofecoxib) and Bextra® (valdecoxib)]. Unlike the effects of aspirin on the action and synthesis activities of COX-2, this latter class of drug does not induce the synthesis of anti-inflammatory lipids. In fact the cardiac benefits of low-dose aspirin are negated when taken along with COX-2 specific inhibitors such as Celebrex.
Another class of anti-inflammatory drug, the corticosteroidal drugs, act to inhibit PLA2, thereby inhibiting the release of arachidonate from membrane phospholipids and the subsequent synthesis of eicosanoids.
Table of Major Biologically Relevant Eicosanoids and Their Functions
Eicosanoid | Major site(s) of synthesis | Major Biological Activities |
LXA4 | platelets, endothelial cells, mucosal epithelial cells and other leukocytes via inteactions with PMNs | reduce PMN and eosinophil infiltration to sites of inflammation, stimulate nonphlogistic (non-inflammatory-inducing) monocyte recruitment, stimulate macrophage phagocytosis of apoptotic PMNs, block IL-8 (chemokine) expression, block TNFα release and actions, stimulate TGF-β action; increases prostacyclin (PGI2 secretion by endothelial cells |
LXB4 | platelets, endothelial cells, mucosal epithelial cells and other leukocytes via interactions with PMNs | same as for LXA4 |
PGD2 | mast cells, eosinophils, brain | inhibits platelet aggregation; induces inflammatory responses at nanomolar concentrations principally by recruiting eosinophils and basophils; induces bronchoconstriction; involved in androgenetic alopecia, inhibitors of PGD2 being studied to treat male pattern baldness |
PGE1 | endothelial cells, smooth muscle | series 1 eicosanoid; functions by binding to both EP2 and EP4; counters effects of PGE2; induces vasodilation and inhibits platelet aggregation; involved in wound healing; alprostadil is drug version of PGE1 formerly used in the treatment of erectile dysfunction |
PGE2 | kidney, spleen, heart, macrophages | series 2 eicosanoid; effects vary depending upon the specific prostaglandin receptor to which it binds (EP1, EP2, EP3, or EP4); binding to the EP3 receptor results in pro-inflammatory effects; binding to the EP4 receptor leads to vasodilation whereas binding to the EP1 receptor results in vasoconstriction; enhancement of platelet aggregation at low physiologic concentration, inhibition at supraphysiologic concentrations |
PGF2α | kidney, spleen, heart | increases vasoconstriction, bronchoconstriction, and smooth muscle contraction |
PGH2 | precursor to thromboxanes A2 and B2, induction of platelet aggregation and vasoconstriction | |
PGI2 | heart, vascular endothelial cells | commonly called prostacyclin; inhibits ADP-induced platelet aggregation; inhibits leukocyte aggregation; decreases T-cell proliferation and lymphocyte migration and secretion of IL-1α and IL-2; induces vasodilation and production of cAMP |
TXA1 | functions in opposition to TXA2; induces vasodilation and inhibits platelet aggregation | |
TXA2 | platelets | induces irreversible platelet aggregation; functions as a vascular smooth muscle cells growth factor; enhances vasoconstriction; increases lymphocyte proliferation; induces bronchoconstriction |
TXB2 | platelets | induces vasoconstriction |
LTB4 | monocytes, basophils, neutrophils, eosinophils, mast cells, epithelial cells | inducer of leukocyte chemotaxis and aggregation; increases vascular permeability; increases T-cell proliferation and secretion of INF-γ, IL-1, and IL-2; promotes adhesion of neutrophils to the vascular endothelium; enhances expression of MCP-1 (monocyte chemoattractant protein 1) which in turn attracts other immune cells to the area |
LTC4 | monocytes and alveolar macrophages, basophils, eosinophils, mast cells, epithelial cells | microvascular vasoconstrictor; enhances vascular permeability; enhances bronchoconstriction and secretion of INF-γ; recruitment of leukocytes to sites of inflammation; enhances mucus secretions in gut and airway |
LTD4 | monocytes and alveolar macrophages, eosinophils, mast cells, epithelial cells | same as LTC4 |
LTE4 | mast cells and basophils | same as LTC4 |
Epoxide Metabolites of Arachidonic Acid
Cytochrome P450 (CYP) epoxygenases are members of the CYP2C and CYP2J families of cytochrome P450 enzymes. The CYP2C and CYP2J enzymes are located in the endoplasmic reticulum (ER).
These epoxygenases convert arachidonic acid to four regioisomers (positional isomers) of epoxyeicosatrienoic acid (EET; also indicated as EpETrE). These EET are 5,6-EET, 8,9-EET, 11,12-EET, and 14,15-EET.
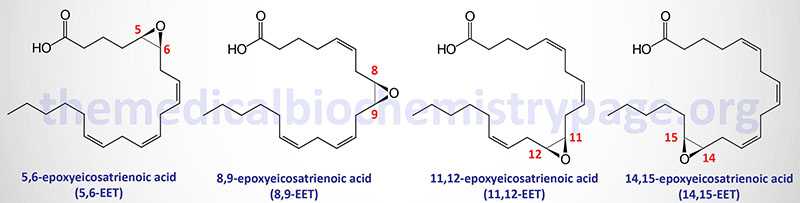
The CYP2J2 encoded enzyme converts arachidonic acid to 5,6-EET. The CYP2C9 and CYP2J2 encoded enzymes can both convert arachidonic acid to 8,9-EET. The CYP2C8, CYP2C9, and CYP2J2 encoded enzymes can all convert arachidonic acid to 11,12-EET. The CYP2C8, CYP2C9, and CYP2J2 encoded enzymes can all convert arachidonic acid to 14,15-EET.
Although the CYP epoxygenases can generate all four of these regioisomers, 11,12-EET and 14,15-EET are the predominant compounds produced in humans. Each of these EET has been shown to function as autocrine and paracrine mediators.
The arachidonic acid substrate for these CYP epoxygenases is released from membrane phospholipids though the action of the Ca2+-dependent type IV phospholipase A2 (PLA2) which is encoded by the PLA2G4A gene.
CYP epoxygenases also convert the omega-3 PUFA, eicosapentaenoic acid (EPA) and docosahexaenoic acid (DHA), to the vasoactive and anti-inflammatory epoxy-derivatives, of which at least five regioisomers of EPA epoxides have been identified and six from DHA. In addition, the ethanolamide (EA) derivatives of EPA and DHA are converted to epoxides by CYP epoxygenases. The CYP epoxygenases are also involved in the formation of anandamide (an endocannabinoid) containing either an 11,12-epoxide or a 14,15-epoxide.
The EET produce vascular relaxation through their ability to activate large-conductance voltage- and Ca2+-activated K+ channels (KCa1.1; also known as BKCa) in vascular smooth muscle cells. Endothelial cells express CYP2C9 and CYP2J2 and these enzymes represent the major source of the EET in the vasculature. The ability of 14,15-EET to induce vasodilation appears to be the result of the compound acting as a selective endogenous antagonist of the thromboxane receptor (TP). In addition, EET have anti-inflammatory effects in the vasculature, they promote angiogenesis, and they protect the heart (myocardium) and brain during ischemic episodes.
The functions attributable to a particular EET can be complicated by the fact that the enantiomeric distribution, and function, of the same regioisomer produced by two different CYP epoxygenases can be markedly different. As an example, when 11,12-EET is produced by CYP2C8, 82% of it is the R/S enantiomer. However, when this same EET is produced by CYP2C10, 69% is the S/R enantiomer. Functionally there is significance to these differences in EET stereoisomers. For example, 11(R),12(S)-EET relaxes small renal arteries but 11(S),12(R)-EET does not.
Many EET actions appear to be initiated by EET binding to a membrane receptor that activates ion channels and intracellular signal transduction pathways. Definitive plasma membrane receptors for the EET have yet to be defined but some studies suggest the free fatty acid receptor 1 (FFAR1; formerly identified as GPR40) exhibits affinity for some of the EET. However, evidence indicates that the EET are also taken up by cells. Cellular uptake of EET appears to be a function for members of the fatty acid binding protein (FABP) family.
EET are incorporated into phospholipids and they bind to cytosolic proteins and nuclear receptors. These data suggest that some functions attributable to EET may occur through direct interaction with intracellular effector systems.
The majority of the EET in the plasma is present in the phospholipids associated with LDL. Although small amounts of 8,9-, 11,12-, and 14,15-EET are present in the liver and kidney, in addition to the plasma, 14,15-EET the most abundant of these various EET. Within membrane phospholipid of the liver and kidney the EET are predominantly localized to the sn-2 position of phosphatidylcholine (PC), phosphatidylethanolamine (PE), and phosphatidylinositol (PI). The main enantiomers in these phospholipids are 8(S),9(R)-EET, 11(S),12(R)-EET, and 14(R),15(S)-EET.
The binding of EET to plasma membrane receptors is most likely via members of the Gs-coupled GPCR family. This is based upon observations that EET activate adenylate cyclase and, as a result, PKA. Following activation of Gs-coupled pathways, EET have been shown to activate intracellular signal transduction pathways involving inhibitor of nuclear factor-κB (IκB) and the IκB-activated kinase (IKK), MAP kinases, and PI3K and AKT/PKB. The activation of these various signal transduction processes ultimately lead to inhibition of inflammation, angiogenesis, increase cell growth, vascular relaxation, bronchodilation, and anti-migratory fibrinolysis, to name a few.
Within the brain, EET are synthesized by astrocytes through a mechanism linked to metabotropic glutamate receptors (mGluR) and adenosine A2B receptors. These actions couple EET to neurovascular remodeling. Within the brain the EET produce anti-nociception (inhibition of pain) effects by activating β-endorphin and met-enkephalin which in turn interact with μ- and δ-opioid receptors. In addition, EET have been shown to reduce brain ischemia and infarct size in stroke.
Epoxide hydrolases convert EET to dihydroxyeicosatrienoic acids (DHET) which represents an important reaction for the attenuation of EET functions. Humans express four epoxide hydrolase genes, identified as EPHX1-EPHX4, that encode enzymes of the α/β hydrolase fold (ABHD) enzyme family. The EPHX2 encoded enzyme is commonly called soluble epoxide hydrolase (sEH). The EPHX1 encoded enzyme (commonly called microsomal epoxide hydrolase, mEH) and the EPHX3 encoded enzyme (also identified as ABHD9) also carry out the metabolism of PUFA-derived epoxides.
Soluble Epoxide Hydrolase (sEH)
The EPHX2 encoded sEH enzyme possesses two independently functioning domains. The N-terminal domain is a phosphatase and the C-terminal domain harbors the epoxide hydrolase activity. The functional sEH enzyme is a homodimer. The products of sEH action on lipid epoxides are the corresponding diols.
The EPHX2 gene is located on chromosome 8p21.2–p21.1 and is composed of 19 exons that generate 12 alternatively spliced mRNAs encoding 11 distinct protein isoforms.
The level of expression of the EPHX2 gene and the level of sEH activity, as well as the concentrations of sEH-produced lipid metabolites, are elevated in many tissues in obese states. In laboratory animals the inhibition of sEH activity has been shown to attenuate the development of various obesity-induced disorders, such as insulin resistance, the metabolic syndrome, and hepatic steatosis. Targeting sEH activity could hold promise in the treatment of obesity and obesity-related disorders. Pharmacological inhibitors of sEH are already undergoing evaluation in numerous human clinical trials.
Omega (ω) Hydroxylated Metabolite (20-HETE) of Arachidonic Acid
The microsomal (endoplasmic reticulum, ER) pathway of fatty acid ω-oxidation involves a family of ω-hydroxylases that are members of the cytochrome P450 (CYP) family of enzymes. These enzymes are abundant in the liver and kidneys. Specifically, the human ω-hydroxylase enzymes are members of the CYP4A and CYP4F families that preferentially hydroxylate the terminal methyl group of C10–C26 length fatty acids.
CYP4A11 was the first ω-hydroxylase characterized. CYP4A11 utilizes NADPH and O2 to introduce a hydroxyl (–OH) group to the ω-CH3– of several fatty acids including lauric (12:0), myristic (14:0), palmitic (16:0), oleic (18:1), and arachidonic acid (20:4).
The formation of ω-hydroxylated arachidonic acid, identified as 20-hydroxyeicosatetraenoic acid (20-HETE), by CYP4A11 plays an important role in various pathologies. 20-HETE is involved in the activation of pro-inflammatory pathways, and the signaling pathways leading to hypertension. Both of these pathologic states contribute to the development of obesity, type 2 diabetes, and cardiovascular disease.
Two additional CYP4 family member enzymes, CYP4F2 and CYP4F3B, convert arachidonic acid to 20-HETE.
The function of 20-HETE is exerted through its binding to a specific GPCR identified as GPR75. GPR75 is coupled to a Gq-type G-protein and thus, its activation results in increased IP3 and DAG production. The increased production of IP3 results in release of ER stored Ca2+ and activation of various Ca2+-mediated signaling cascades.
With respect to the vasculature, the activation of GPR75 by 20-HETE in vascular smooth muscle cells leads to inhibition of the large conductance calcium- and voltage-activated potassium channel (designated KCa1.1 and encoded by the KCNMA1 gene). The KCNMA1 encoded potassium channel is also known as MaxiK or BK (big potassium). The inhibition of the KCNMA1 channel underlies the hypertension-inducing effects of 20-HETE. Indeed, polymorphisms in the CYP4A11 gene are associated with hypertension in certain populations, particular Asian populations.
Hepoxilins
The hepoxilins (designated Hx) are derived through metabolism of arachidonic acid. These lipids contain an epoxide group located between C-11 and C-12 that is oriented in the S,S configuration. There are two hepoxilins that are formed via enzymatic metabolism from 12(S)-hydroperoxy eicosatetraenoic acid [12(S)-HpETE] which itself is derived from arachidonic acid through the action of 12-lipoxygenase (12-LOX; encoded by the ALOX12 gene). These hepoxilins are identified as HxA3 and HxB3. As indicated above, the ALOX12 encoded enzyme is also identified as 12(S)-LOX. The conversion of 12(S)-HpETE to HxB3 is catalyzed by the ALOXE3 encoded enzyme which is commonly identified as E-LOX.
Hepoxilin synthesis can also proceed via the actions of the ALOX12B encoded enzyme which is commonly referred to as 12(R)-LOX. The action of the ALOX12B encoded enzyme generates 12(R)-HpETE from arachidonic acid which is then converted to HxA3 via the actions of the ALOXE3 encoded enzyme, E-LOX.
In addition to the enzymatic generation of HxA3 and HxB3, these hepoxilins can be non-enzymatically generated from both 12(S)- and 12(R)-HpETE. The catalysts for these non-enzymatic conversions have been shown to be hemin or hemoglobin.
The hepoxilins are unstable and are metabolized to trihydroxy products (TrXA3 and TrXB3) through epoxide ring opening by soluble epoxide hydrolases (sEH). These trihydroxy derivatives do not exhibit any biological activity. The epoxide moiety of HxA3 can be opened by glutathione S-transferases which yields biologically active peptido conjugates such as HxA3-C which exerts effects in the brain and on platelets.
Numerous biological activities have been attributed to HxA3 such as the stimulation of insulin secretion in a glucose-dependent manner which is likely to be mediated by mobilization of calcium ions into the cytosol of pancreatic β-cells. Experiments with other cell types has demonstrated that HxA3 induces the mobilization of Ca2+ from intracellular stores and also increases intracellular Ca2+ by enhancing uptake across the plasma membrane. HxA3 has also been shown to exert effects on inflammatory responses by acting as a chemotactic factor for neutrophils. The hepoxilins have also been shown to potentiate the vasoconstrictive actions of norepinephrine on vascular smooth muscle cells.
Arachidonic Acid-Derived Isoprostanes (IsoP)
The isoprostanes (IsoP) are prostaglandin-like molecules that are generated by the free radical-induced peroxidation of arachidonic acid. The first IsoP species to be characterized were shown to be isomeric to prostaglandin F2α (PGF2α) and were therefore, termed F2-isoprostanes (F2-IsoP). Isoprostanes are predominantly incorporated into phospholipids in cellular membranes.
The mechanism of the formation of the F2-IsoP from arachidonic acid involves the abstraction of a bisallylic hydrogen atom and the addition of molecular oxygen forming a peroxyl radical. These peroxyl radicals undergo a 5-exo cyclization followed by the addition of a second molecule of oxygen. The resultant molecules are PGG2-like in their structure. The bicycloendoperoxide intermediates are unstable and are reduced to the F2-IsoP. The mechanism of IsoP formation allows for four F2-IsoP regioisomers to be generated. Each of these four regioisomers is comprised of eight racemic diastereomers such that a total of 64 compounds can be generated. The four regioisomer classes are named according to the carbon number on which the side chain hydroxyl group is attached such that there is the 5-series F2-IsoP (classified as type VI), the 8-series F2-IsoPs (classified as type IV), the 12-series F2-IsoP (classified as type V), and the 15-series F2-IsoP (classified as type III).
Isoprostanes that are isomeric to PGD2 and PGE2 are also formed in competition with the formation of the F2-IsoP. The PGD2 isomeric isoprostanes are identified as D2-IsoP and those isomeric to PGE2 are identified as E2-IsoP. The D2-IsoP and E2-IsoP compounds are unstable and will dehydrate to yield the A2-IsoP and J2-IsoP compounds, respectively.
The most well studied isoprostanes are the F2-IsoP. One F2-IsoP in particular, 15-F2τ-IsoP, has been shown to exert its effects by interacting with the thromboxane receptor. The isoprostanes have also been shown to exert biological activity by binding to PGE2 receptors and the PGF2α receptor. The ultimate biological activity of a given IsoP varies depending upon the receptor to which it binds. For example, 15-F2τ-IsoP and 15-E2τ-IsoP have been shown to induce vasoconstriction when binding to the thromboxane receptor they induce vasodilation upon binding to PGE2 receptors.
Although formation of the multitude of isoprostanes occurs naturally, their production is associated with oxidative stress in various disease states in humans. The concentration of F2-IsoP found in the urine has been shown to be increased under a variety of oxidative stress conditions such as in overweight and obese individuals and in patients undergoing cancer chemotherapy. Ischemia-reperfusion injury is a major concern during coronary artery bypass grafting surgery, cardiopulmonary bypass surgery, and organ transplantation. The levels of F2-IsoP increase following these surgical procedures and interestingly, the pretreatment of patients who will be undergoing these types of procedures with aspirin has been shown to reduce the production of the F2-IsoP.