Last Updated: September 16, 2024
Chemical Nature of the Amino Acids
All peptides and polypeptides are polymers of α-amino acids. There are 20 α-amino acids that are relevant to the make-up of mammalian proteins (see below). Several other amino acids are found in the body free or in combined states (e.g. not associated with peptides or proteins). These non-protein associated amino acids perform specialized functions such as citrulline and ornithine in the disposal of waste nitrogen via the urea cycle. Several of the amino acids found in proteins also serve functions distinct from the formation of peptides and proteins, e.g., tyrosine in the formation of thyroid hormones or glutamate acting as a neurotransmitter.
The α-amino acids in peptides and proteins (excluding proline) consist of a carboxylic acid (–COOH) and an amino (–NH2) functional group attached to the same tetrahedral carbon atom. This carbon is the α-carbon. Distinct R-groups, that distinguish one amino acid from another, also are attached to the alpha-carbon (except in the case of glycine where the R-group is hydrogen). The fourth substitution on the tetrahedral α-carbon of amino acids is hydrogen.
Table of α-Amino Acids Found in Proteins
Amino Acid | Symbol | Structure* | pK1 (COOH) | pK2 (NH2) | pK R Group |
---|---|---|---|---|---|
Amino Acids with Aliphatic R-Groups | |||||
Glycine | Gly (G) | ![]() | 2.4 | 9.8 | |
Alanine | Ala (A) | ![]() | 2.4 | 9.9 | |
Valine | Val (V) | ![]() | 2.2 | 9.7 | |
Leucine | Leu (L) | ![]() | 2.3 | 9.7 | |
Isoleucine | Ile (I) | ![]() | 2.3 | 9.8 | |
Non-Aromatic Amino Acids with Hydroxyl R-Groups | |||||
Serine | Ser (S) | ![]() | 2.2 | 9.2 | ≈13 |
Threonine | Thr (T) | ![]() | 2.1 | 9.1 | ≈13 |
Amino Acids with Sulfur-Containing R-Groups | |||||
Cysteine | Cys (C) | ![]() | 1.9 | 10.8 | 8.3 |
Methionine | Met (M) | ![]() | 2.1 | 9.3 | |
Acidic Amino Acids and their Amides | |||||
Aspartic Acid | Asp (D) | ![]() | 2.0 | 9.9 | 3.9 |
Asparagine | Asn (N) | ![]() | 2.1 | 8.8 | |
Glutamic Acid | Glu (E) | ![]() | 2.1 | 9.5 | 4.1 |
Glutamine | Gln (Q) | ![]() | 2.2 | 9.1 | |
Basic Amino Acids | |||||
Arginine | Arg (R) | ![]() | 1.8 | 9.0 | 12.5 |
Lysine | Lys (K) | ![]() | 2.2 | 9.2 | 10.8 |
Histidine | His (H) | ![]() | 1.8 | 9.2 | 6.0 |
Amino Acids with Aromatic Rings | |||||
Phenylalanine | Phe (F) | ![]() | 2.2 | 9.2 | |
Tyrosine | Tyr (Y) | ![]() | 2.2 | 9.1 | 10.1 |
Tryptophan | Trp (W) | ![]() | 2.4 | 9.4 | |
Imino Acids | |||||
Proline | Pro (P) | ![]() | 2.0 | 10.6 |
*Backbone of the amino acids is red, R-groups are black
Amino Acid Classifications
Each of the 20 α-amino acids found in proteins can be distinguished by the R-group substitution on the α-carbon atom. There are two broad classes of amino acids based upon whether the R-group is hydrophobic or hydrophilic.
The hydrophobic amino acids tend to repel the aqueous environment and, therefore, reside predominantly in the interior of proteins. This class of amino acids does not ionize nor participate in the formation of H-bonds. The hydrophilic amino acids tend to interact with the aqueous environment, are often involved in the formation of H-bonds and are predominantly found on the exterior surfaces proteins or in the reactive centers of enzymes.
Acid-Base Properties of the Amino Acids
The α-COOH and α-NH2 groups in amino acids are capable of ionizing (as are the acidic and basic R-groups of the amino acids). As a result of their ionizability the following ionic equilibrium reactions may be written:
R-COOH ↔ R-COO– + H+
R-NH3+ ↔ R-NH2 + H+
The equilibrium reactions, as written, demonstrate that amino acids contain at least two weakly acidic groups. However, the carboxyl group is a far stronger acid than the amino group. At physiological pH (around 7.4) the carboxyl group will be unprotonated and the amino group will be protonated. An amino acid with no ionizable R-group would be electrically neutral at this pH. This species is termed a zwitterion.
Like typical organic acids, the acidic strength of the carboxyl, amino and ionizable R-groups in amino acids can be defined by the association constant, Ka or more commonly the negative logarithm of Ka, the pKa. The net charge (the algebraic sum of all the charged groups present) of any amino acid, peptide or protein, will depend upon the pH of the surrounding aqueous environment. As the pH of a solution of an amino acid or protein changes so too does the net charge. This phenomenon can be observed during the titration of any amino acid or protein. When the net charge of an amino acid or protein is zero the pH will be equivalent to the isoelectric point: pI.
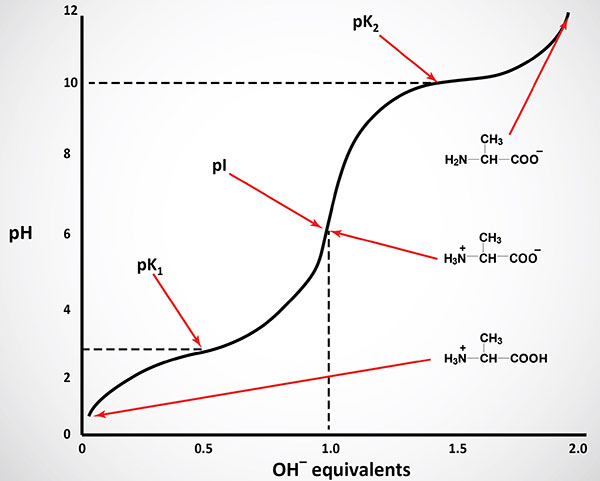
Functional Significance of Amino Acid R-Groups
In solution it is the nature of the amino acid R-groups that dictate structure-function relationships of peptides and proteins. The hydrophobic amino acids will generally be encountered in the interior of proteins shielded from direct contact with water. Conversely, the hydrophilic amino acids are generally found on the exterior of proteins as well as in the active centers of enzymatically active proteins. Indeed, it is the very nature of certain amino acid R-groups that allow enzyme reactions to occur.
The imidazole ring of histidine allows it to act as either a proton donor or acceptor at physiological pH. Hence, it is frequently found in the reactive center of enzymes. Equally important is the ability of histidines in hemoglobin to buffer the H+ ions from carbonic acid ionization in red blood cells. It is this property of hemoglobin that allows it to exchange O2 and CO2 at the tissues or lungs, respectively.
The primary alcohol of serine and threonine as well as the thiol (–SH) of cysteine allow these amino acids to act as nucleophiles during enzymatic catalysis. Additionally, the thiol of cysteine is able to form a disulfide bond with other cysteines:
Cysteine-SH + HS-Cysteine ↔ Cysteine-S-S-Cysteine
This simple disulfide is identified as cystine. The formation of disulfide bonds between cysteines present within proteins is important to the formation of active structural domains in a large number of proteins. Disulfide bonding between cysteines in different polypeptide chains of oligomeric proteins plays a crucial role in ordering the structure of complex proteins, e.g. the insulin receptor.
Optical Properties of the Amino Acids
A tetrahedral carbon atom with 4 distinct constituents is said to be chiral. The one amino acid not exhibiting chirality is glycine since its ‘”R-group” is a hydrogen atom. Chirality describes the handedness of a molecule that is observable by the ability of a molecule to rotate the plane of polarized light either to the right (dextrorotatory) or to the left (levorotatory). All of the amino acids in proteins exhibit the same absolute steric configuration as L-glyceraldehyde. Therefore, they are all L-α-amino acids. D-amino acids are never found in proteins, although they exist in nature. D-amino acids are often found in polypeptide antibiotics.
The aromatic R-groups in amino acids absorb ultraviolet light with an absorbance maximum in the range of 280nm. The ability of proteins to absorb ultraviolet light is predominantly due to the presence of the tryptophan which strongly absorbs ultraviolet light.
The Peptide Bond
Peptide bond formation is a condensation reaction leading to the polymerization of amino acids into peptides and proteins. Peptides are small consisting of few amino acids. A number of hormones and neurotransmitters are peptides. Additionally, several antibiotics and antitumor agents are peptides. Proteins are polypeptides of greatly divergent length. The simplest peptide, a dipeptide, contains a single peptide bond formed by the condensation of the carboxyl group of one amino acid with the amino group of the second with the concomitant elimination of water. The presence of the carbonyl group in a peptide bond allows electron resonance stabilization to occur such that the peptide bond exhibits rigidity not unlike the typical –C=C– double bond. The peptide bond is, therefore, said to have partial double-bond character.
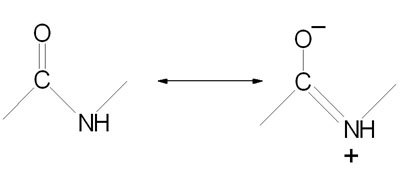
Non-Protein Amino Acids
Human cells produce and utilize several amino acids that are not involved in the synthesis of proteins. The more physiologically relevant non-protein amino acids include α-amino acids, non-α-amino acids, and the β-alanyl dipeptides.
The non-protein α-amino acids include ornithine, citrulline, argininosuccinate, thyroxine (T4), triiodothyronine (T3), homocysteine, S-adenosylmethionine (SAM), 3,4-dihydroxyphenylalanine (L-DOPA), and creatinine. Ornithine, citrulline, and argininosuccinate are intermediates in the urea cycle. Thyroxine (T4) and triiodothyronine (T3) are the thyroid hormones. Homocysteine is an intermediate in the methionine cycle, a pathway of methionine catabolism and cysteine synthesis, and excess production results in homocystinemia/homocystinuria. S-Adenosylmethionine (SAM; also denoted AdoMet) is produced in the methionine cycle and is utilized as the major donor of methyl groups in hundreds of methylation reactions that include the epigenetic modification of DNA and histone proteins as well as membrane lipid modification and neurotransmitter synthesis. 3,4-Dihydroxyphenylalanine is an intermediate in the catecholamine biosynthesis pathway. Creatinine is the non-enzymatic byproduct of creatine which is produced primarily in skeletal muscle as an energy storage molecule, creatine phosphate.
The non-α-amino acids include β-alanine, β-aminoisobutyric acid (BAIBA), γ-aminobutyric acid (GABA), taurine (2-aminoethane-sulfonic acid), and δ-aminolevulinic acid (ALA). Beta-alanine is a byproduct of the catabolism of pyrimidine nucleotides as well as from the catabolism of the β-alanyl dipeptides, carnosine and anserine. Beta-aminoisobutyric acid (BAIBA) exists in two enantiomeric forms, D-BAIBA and L-BAIBA, the former being derived from the catabolism of thymine and the latter from the catabolism of valine. The major source of BAIBA is exercising skeletal muscle and as such it is considered both as a myokine and as an exerkine. Gamma-aminobutyric acid (GABA) is an inhibitory neurotransmitter. Delta-aminolevulinic acid is an intermediate in the biosynthesis of heme.
Taurine is synthesized from cysteine via a three step reaction pathway or is acquired in the diet. Taurine is utilized in the synthesis of the bile acids and also serves numerous other important functions in the body. Taurine is one of the most abundant amino acids in skeletal muscle, brain, and retina. In the retina taurine functions in photoreceptor development. In the brain taurine serves as a cytoprotectant against stress-related neuronal damage and other pathological conditions. Taurine is also an organic osmolyte involved in the regulation of cell volume. Taurine also plays important roles in the modulation of intracellular free calcium concentration and in the protection from mitochondrial stress.
The β-alanyl dipeptides include carnosine (β-alanyl-L-histidine), homocarnosine (γ-aminobutyryl-L-histidine), and anserine (N-methylcarnosine). Carnosine functions as a major buffer in skeletal muscle to help delay the onset of lactic acid-induced muscle fatigue and failure, as well as enhancing endurance and improving recovery from anaerobic exercise.