Last Updated: September 16, 2024
Major Roles of Biological Lipids
Biological molecules that are insoluble in aqueous solution and soluble in organic solvents are classified as lipids. Lipids in biological systems include fats, sterols, fat soluble vitamins, phospholipids, and triglycerides. The lipids of physiological importance for humans exert the following major functions:
1. They serve as structural components of biological membranes.
2. They provide energy reserves, predominantly in the form of triglycerides (TG; also called triacyglycerols, TAG).
3. Lipids and lipid derivatives serve as biologically active molecules exerting a wide range of functions.
4. Lipophilic bile acids aid in emulsification, digestion, and absorption of dietary lipids as well as being a form of bioactive lipids.
Fatty Acids
Fatty acids fill three major roles in the body:
- as the components of more complex membrane lipids.
- as the major components of stored fat in the form of triglycerides.
- as the precursors for the synthesis of bioactive lipids.
Fatty acids are long-chain hydrocarbon molecules containing a carboxylic acid moiety at one end. The numbering of carbons in fatty acids begins with the carbon of the carboxylate group. At physiological pH, the carboxyl group is readily ionized, rendering a negative charge onto fatty acids in bodily fluids.
Fatty Acid Saturation States
Fatty acids that contain no carbon-carbon double bonds are termed saturated fatty acids; those that contain double bonds are unsaturated fatty acids and fatty acids with multiple sites of unsaturation are termed polyunsaturated fatty acids (PUFA). The numeric designations used for fatty acids come from the number of carbon atoms, followed by the number of sites of unsaturation (e.g., palmitic acid is a 16-carbon fatty acid with no unsaturation and is designated by 16:0).

The melting point of fatty acids increases as the number of carbon atoms increases. In addition, the introduction of sites of unsaturation results in lower melting points when comparing a saturated and an unsaturated fatty acid of the same number of carbon atoms. Saturated fatty acids of less than eight carbon atoms are liquid at physiological temperature, whereas those containing more than ten are solid. As a general rule, oils from vegetables contain many more unsaturated fatty acids and are therefore, liquids at room temperature. In contrast, animal oils contain more saturated fatty acids. The steric geometry of unsaturated fatty acids can also vary such that the acyl groups (or hydrogen atoms) can be oriented on the same side or on opposite sides of the double bond. When the acyl groups (or hydrogen atoms) are both on the same side of the double bond it is referred to as a cis bond, such as is the case for oleic acid (18:1). When the acyl groups (or hydrogen atoms) are on opposite sides the bond is termed trans such as in elaidic acid, the trans isomer of oleic acid.
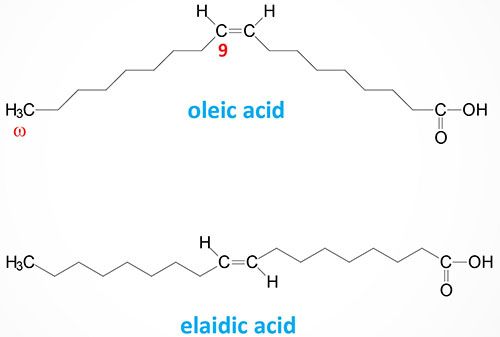
The majority of naturally occurring unsaturated fatty acids exist in the cis-conformation. Trans fatty acids occur in some foods and as byproducts of the process of hydrogenating unsaturated fatty acids to make them solids at room temperature, such as in partially hydrogenated vegetable oils. Diets high in trans fatty acids have been associated with an increased risk of cardiovascular disease and development of the metabolic syndrome and have, therefore, been banned from manufactured food products by most major governments.
The site of unsaturation in a fatty acid is indicated by the symbol Δ and the number of the first carbon of the double bond relative to the carboxylic acid group (–COOH) carbon which is designated carbon #1. For example, palmitoleic acid is a 16-carbon fatty acid with one site of unsaturation between carbons 9 and 10, and is designated by 16:1Δ9.
The majority of fatty acids found in the body are acquired in the diet. However, the lipid biosynthetic capacity of the body (fatty acid synthase and other fatty acid modifying enzymes) can supply the body with all the various fatty acid structures needed.
Two key exceptions to this are the PUFA known as linoleic acid and α-linolenic acid (ALA), containing unsaturation sites beyond carbons 9 and 10 (relative to the α-COOH group). These two fatty acids cannot be synthesized from precursors in the body, and are thus considered the essential fatty acids; essential in the sense that they must be provided in the diet. Since plants are capable of synthesizing linoleic and α-linolenic acid, humans can acquire these fats by consuming a variety of plants or else by eating the meat of animals that have consumed these plant fats.
These two essential fatty acids are also referred to as omega fatty acids. The use of the Greek omega (ω) refers to the end of the fatty acid opposite to that of the –COOH group. Linoleic acid is an omega-6 PUFA and α-linolenic is an omega-3 PUFA (see Table below). The role of PUFAs, such as linoleic and α-linolenic, in the synthesis of biologically important lipids is described briefly below and also in the Synthesis of Fatty Acids page, the Bioactive Lipids and Lipid Sensing Receptors page, and the Bioactive Lipid Mediators of Inflammation page.
Table of Physiologically Relevant Fatty Acids
Numerical Symbol | Common Name and Structure | Comments |
14:0 | Myristic acid![]() | often found attached to the N-term. of plasma membrane-associated cytoplasmic proteins |
16:0 | Palmitic acid![]() | end product of mammalian fatty acid synthesis |
16:1Δ9 | Palmitoleic acid![]() | major monounsaturated fatty acid (MUFA) in humans; omega-7 fatty acid |
18:0 | Stearic acid![]() | |
18:1Δ9 | Oleic acid![]() | major monounsaturated fatty acid (MUFA) in humans; omega-9 fatty acid |
18:2Δ9,12 | Linoleic acid![]() | essential fatty acid omega-6 polyunsaturated fatty acid (PUFA) |
18:3Δ9,12,15 | α-Linolenic acid (ALA)![]() | essential fatty acid omega-3 polyunsaturated fatty acid (PUFA) |
20:4Δ5,8,11,14 | Arachidonic acid![]() | omega-6 polyunsaturated fatty acid (PUFA) precursor for eicosanoid synthesis |
20:5Δ5,8,11,14,17 | Eicosapentaenoic acid (EPA)![]() | omega-3 polyunsaturated fatty acid (PUFA) enriched in fish oils, Krill oil, and cyanobacteria |
22:6Δ4,7,10,13,16,19 | Docosahexaenoic acid (DHA)![]() | omega-3 polyunsaturated fatty acid (PUFA) enriched in fish oils, Krill oil, and cyanobacteria |
Oleic acid (18:1) is the most abundant monounsaturated fatty acid (MUFA) in the human body. Palmitoleic acid (16:1) is also an abundant MUFA in human cells. These two fatty acids represent the majority of MUFA present in membrane phospholipids, triglycerides, and cholesterol esters. The health benefits of oleic acid are broad and profound. Numerous studies have shown that consumption of MUFA is important to maintain low levels of LDL in the blood and is also likely to be associated with the potential for elevated HDL. Another physiologically significant effect of oleic acid is the result of its conversion to oleoylethanolamide (OEA) in the small intestine. OEA has demonstrated effects in the CNS related to the control of appetite and feeding behaviors. For more information on this latter function of oleic acid go the the Bioactive Lipid and Lipid Sensing Receptors page.
Excellent vegetarian and vegan sources of oleic acid are olive oil in which up to 85% of the triglyceride in this oil contains oleic acid. Free oleic acid levels in food grade olive oil are mandated to be less than 2% as more than this makes the oil inedible. Extra virgin olive oils will have less than 0.8% free oleic acid. Other vegetable and nut oils also contain high levels of oleic acid in their triglycerides with canola oil (60%–65%) having the second highest amount compared to olive oil, pecan oil has 60%–75% oleic in triglyceride. Another excellent source of oleic acid (43%) is argan oil (from the argan tree which is abundant in Morocco). In addition to the presence of oleic acid, argan oil has added benefits in that it is high in numerous antioxidant plant phenolic compounds as well as vitamin A and vitamin E. Peanut oil (35%–70%), sunflower oil (20%–80%), and grape seed oil (15%–20%) are also excellent plant sources of oleic acid. Animal fats are also high in oleic acid with lard and tallow containing 44%–47% in the triglyceride component.
Omega-3, and -6, Polyunsaturated Fatty Acids (PUFAs)
The term omega, as it relates to fatty acids, refers to the terminal carbon atom farthest from the functional carboxylic acid group (–COOH). The designation of a polyunsaturated fatty acid (PUFA) as an omega-3 fatty acid, for example, defines the position of the first site of unsaturation relative to the omega end of that fatty acid. Thus, an omega-3 fatty acid like α-linolenic acid (ALA), which harbors three carbon-carbon double bonds (i.e. sites of unsaturation), has a site of unsaturation between the third and fourth carbons from the omega end (see Figure in Table above).
There are three major omega-3 PUFA that are ingested in foods and used by the body: ALA, eicosapentaenoic acid (EPA), and docosahexaenoic acid (DHA). Once eaten, the body converts ALA to EPA and DHA, the two types of omega-3 fatty acids more readily used by the body and which serve as important precursors for lipid-derived modulators of cell signaling, gene expression, and inflammatory processes.
It is important to denote that when discussing omega-3 fatty acids, their dietary origin is quite important. Omega-3 fats from plants, such as those in flax seed oil, are enriched in ALA. As indicated above, ALA must first be converted to EPA (requiring three independent reactions) and then to DHA (requiring an additional four reactions). Omega-3 fats from fish and krill are enriched in EPA and DHA and thus do not need to undergo the complex conversion steps required of ALA. In addition, the conversion of ALA to EPA and DHA is inefficient in individuals consuming a typical Western diet rich in animal fats.
Most of the omega-6 PUFA consumed in the diet are from vegetable oils and consist of linoleic acid. Linoleic acid is converted to γ-linolenic acid (GLA) in the body. GLA should not be confused with ALA which, as pointed out above, is an essential omega-3 PUFA. GLA is converted to dihomo-γ-linolenic acid (DGLA) and then to arachidonic acid as shown in the Eicosanoid Metabolism: Prostaglandins, Thromboxanes, Leukotrienes, and Lipoxins page. Due to the limited activity of the Δ5-desaturase most of the DGLA formed from GLA is inserted into membrane phospholipids at the same C-2 position as for arachidonic acid. GLA can be ingested from several plant-based oils including evening primrose oil, borage oil, and black currant seed oil.
Additional Important Omega Fatty Acids
In the consideration of biologically significant fatty acids, the omega-3 and omega-6 fatty acids, although highly clinically significant, should not completely overshadow the facts that there are two additional omega fats of clinical significance. These additional fatty acids are the monounsaturated fatty acids (MUFA), palmitoleic acid (16:1) and oleic acid (18:1). Palmitoleic acid is an omega-7 MUFA and oleic acid is an omega-9 MUFA.
Palmitoleic acid is likely to be a potent anti-aging fat. Experimental and clinical studies have shown that palmitoleic acid exerts many of its beneficial effects via activation of the master regulatory enzyme called AMP-activated protein kinase, AMPK.
Oleic acid serves as the precursor for the potent bioactive lipid ethanolamide, oleoylethanolamide (OEA). OEA exerts potent effects within the central nervous system to control feeding behavior and in the pancreas to increase glucose-stimulated insulin secretion (GSIS).
Basic Structure of Triglycerides
Triglycerides, which represent the major storage form of lipid in the human body, are composed of a glycerol backbone to which three fatty acids are esterified. The major sites of triglyceride synthesis are adipose tissue and liver, but many other tissues can also synthesize these lipid molecules.
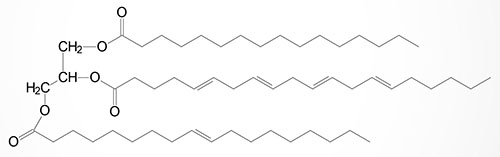
Basic Structure of Phospholipids
The basic structure of phospholipids is very similar to that of the triglycerides except that C–3 (sn3)of the glycerol backbone is esterified to phosphoric acid. The building block of the phospholipids is phosphatidic acid. Substitutions that can be added to phosphatidic acid include ethanolamine (phosphatidylethanolamines, PE), choline (phosphatidylcholines, PC: also called lecithins), serine (phosphatidylserines, PS), glycerol (phosphatidylglycerols, PG), myo-inositol (phosphatidylinositols, PI: these compounds can have a variety in the numbers of inositol alcohols that are phosphorylated generating polyphosphatidylinositols), and phosphatidylglycerol (diphosphatidylglycerols, DPG; more commonly known as cardiolipins). See the Synthesis of Phospholipids page for images of the various phospholipid classes.
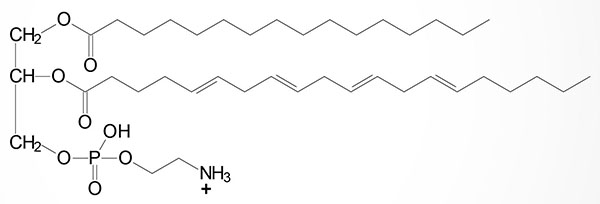
Basic Structure of Plasmalogens
Plasmalogens are complex membrane lipids that resemble phospholipids, principally phosphatidylcholine. The major difference is that the fatty acid at C–1 (sn1) of glycerol contains either an O-alkyl (–O–CH2–) or O-alkenyl ether (–O–CH=CH–) species. An O-alkenyl ether species containing phosphoethanolamine is shown in the Figure although other phospholipid substitutions can be found such as choline or serine.
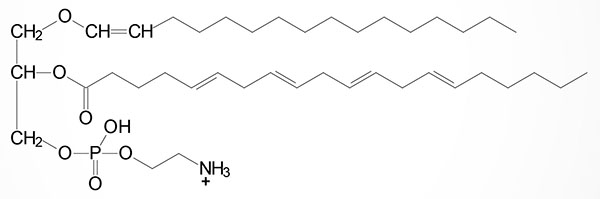
One of the most potent plasmalogens is platelet activating factor (PAF: 1-O-1′-enyl-2-acetyl-sn-glycero-3-phosphocholine) which is a choline plasmalogen in which the C–2 (sn2) position of glycerol is esterified with an acetyl group instead of a long chain fatty acid.
PAF functions as a mediator of hypersensitivity, acute inflammatory reactions and anaphylactic shock. PAF is synthesized in response to the formation of antigen-IgE complexes on the surfaces of basophils, neutrophils, eosinophils, macrophages and monocytes. The synthesis and release of PAF from cells leads to platelet aggregation and the release of serotonin from platelets. PAF also produces responses in liver, heart, smooth muscle, and uterine and lung tissues.
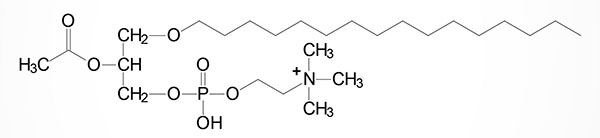
Basic Structure of Sphingolipids
Sphingolipids are composed of a backbone of sphingosine which is derived itself from glycerol. Sphingosine is N-acetylated by a variety of fatty acids generating a family of molecules referred to as ceramides. Sphingolipids predominate in the myelin sheath of nerve fibers. Sphingomyelin is an abundant sphingolipid generated by transfer of the phosphocholine moiety of phosphatidylcholine to a ceramide, thus sphingomyelin is a unique form of a phospholipid.
The other major class of sphingolipids (besides the sphingomyelins) are the glycosphingolipids generated by substitution of carbohydrates to the sn1 carbon of the sphingosine backbone of a ceramide. There are 4 major classes of glycosphingolipids:
Cerebrosides: contain a single moiety, principally galactose, termed a galactocerebroside or galactosylceramide.
Sulfatides: sulfuric acid esters of galactocerebrosides.
Globosides: contain two or more sugars.
Gangliosides: similar to globosides except also contain sialic acid (N-acetylneuraminic acid, NANA).
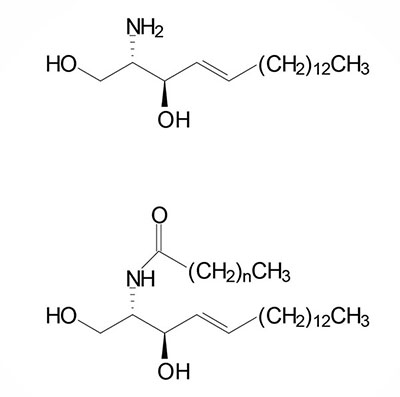
Eicosanoids
The eicosanoids (from Ancient Greek eíkosi for 20) are a family of biologically active lipids composed of twenty carbon atoms that are derived from the polyunsaturated fatty acid (PUFA), arachidonic acid. Arachidonic acid is a 20 carbon fatty acid containing four sites of unsaturation (20:4).
Details of the synthesis and biological activities of the eicosanoids are covered in the Eicosanoid Metabolism: Prostaglandins, Thromboxanes, Leukotrienes, and Lipoxins page. The eicosanoids primarily consist of the prostaglandins (PG), thromboxanes (TX), leukotrienes (LT), and lipoxins (LX). The PG and TX are collectively identified as prostanoids.
Oxylipins
Oxylipins (oxygenated metabolites) are a large family of bioactive lipid molecules that are derived from omega-6 and omega-3 PUFA which includes the essential fatty acids, linoleic acid (omega-6) and linolenic acid (omega-3). The various oxylipins are synthesized via the actions of cyclooxygenases, lipoxygenases, and oxidases of the cytochrome P450 (CYP) family of enzymes. More details on the oxylipin family of bioactive lipids are in the Bioactive Lipids and Lipid Sensing Receptors page.
The prostaglandins, thromboxanes, leukotrienes, and lipoxins all belong to the oxylipin family of bioactive lipids. In addition to the four major types of oxylipins derived from arachidonic acid, additional bioactive oxylipin family lipids that are derived from arachidonic acid, include the resolvins, protectins (also called neuroprotectins in the brain), maresins, mono- and dihydroxy fatty acids, epoxy fatty acids, eoxins, and hepoxilins.
Abundant monohydroxy fatty acids in humans include the arachidonic acid derivatives, 12- and 15-hydroxyeicosatetraenoic acid (12-HETE and 15-HETE) and the linoleic acid derivatives; 9- and 13-hydroxyotadecadienoic acid (9-HODE and 13-HODE).
The dihydroxy fatty acids are derived via the actions of soluble epoxide hydrolase (sEH; encoded by the EPHX2 gene) on lipid epoxides. For example the linoleic acid derived epoxide, 9,10-epoxyoctadecenoic acid (9,10-EpOME; coronaric acid, also known as leukotoxin) is converted to 9,10-dihydroxyoctadecamonoenoic acid (9,10-DiHOME) via the actions of sEH.